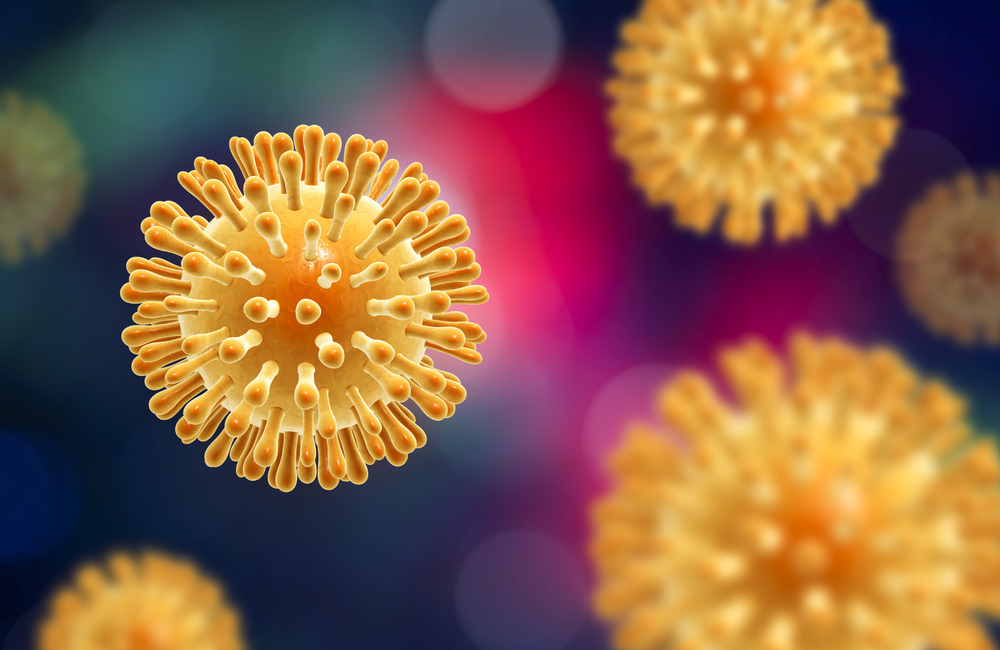
Key points
- HIV can hide in immune cells for many years, but can reveal itself at any time to produce virus.
- These cells (known as the latent reservoir) remain the major barrier to cure.
- HIV cure research is focused on eliminating the HIV reservoir (complete cure), or controlling virus released from the reservoir (functional cure).
- The ‘repair and replace’ strategy aims to remove and replace all infected cells from the body.
- The ‘kick and kill’ approach kicks HIV out of its hiding place, whereas ‘block and lock’ aims to lock HIV in its hiding place.
- Immunotherapies, such as vaccines, aim to enhance the immune system to control or eliminate HIV.
- The search for an HIV cure continues and may require combinations of these approaches.
So far, HIV has proved almost impossible to eradicate from the human body because the virus integrates into long-lived immune system cells and remains dormant in those cells for many years. In most viral infections the immune system recognises infected cells and kills those cells. In a few viral infections, such as herpes simplex (the cause of genital herpes) and HIV, a virus hides itself from the immune system or infects cells that are difficult for the immune system to reach.
The HIV reservoir
HIV mainly infects CD4+ T-lymphocytes, dendritic cells and macrophages. These are immune system cells responsible for recognising pathogens and clearing them. HIV integrates into the DNA of these cells. Most infected cells are short-lived, and if antiretroviral therapy (ART) stops HIV replication they soon die.
Some immune system cells, however, become dormant until they are activated to respond to a pathogen they have already seen and are sensitised to. These are central memory CD4 cells. They are especially long-lasting and may survive into advanced old age.
When a central memory CD4 cell is activated, new copies of HIV are produced, which in turn activate other cells. This is what happens in most cases if people stop taking their ART. When treatment is stopped, HIV rapidly rebounds to pre-treatment levels.
These cells are described as latently infected and form what is known as the HIV reservoir. Reservoir cells are concentrated in the lymph nodes, the gut and the spleen but may also be found in the brain, the kidneys and in other tissues.
Scientists are working on tests to identify and count all HIV-infected cells in the body, as one of the barriers to an HIV cure is that scientists are unsure about which tissues in the body contain HIV-infected cells and so do not know if they are checking for eradication of the virus in all the right places. More reliable tests are needed too. For instance, although many cells may contain HIV DNA, it’s not clear that this DNA always forms an intact viral sequence able to trigger production of new HIV.
Women appear to have lower levels of intact viral sequences and HIV takes longer to rebound when women stop treatment. The female sex hormone oestrogen may limit the HIV reservoir in women. More research is needed to find out if different cure strategies are needed for men and women.
Recent estimates suggest that between 100,000 and 10 million CD4 T-cells out of 200 billion CD4 cells are latently infected and form the reservoir of HIV in the body. But HIV DNA that can give rise to new virions may also be found in other cells and scientists are working on tests to identify and count all HIV-infected cells in the body.
The reservoir of HIV-infected cells becomes established within days of HIV entering the body. There is some evidence that treatment started very soon after infection can limit the size of the reservoir, especially in infants, and this may improve the chances of curing HIV in the future.
Latently infected cells are only detectable by ultrasensitive experimental tests. They are not detected by the viral load tests used to monitor treatment.
Potential cure strategies
Several strategies are being explored to cure HIV.
One strategy is to seek complete eradication of HIV from the body. This has sometimes been called a sterilising cure, meaning that all HIV is eliminated, but as this has unpleasant connotations, researchers and activists now tend simply to talk about elimination, a complete cure or simply a cure. This goal may be difficult to achieve without a better understanding of how HIV persists in the body undetected and untouched by the immune system.
Another strategy is to achieve a functional cure, where HIV is not eliminated from the body but is kept under control by the immune system without the need for antiretroviral treatment or other ongoing medication. This goal is sometimes called long-term viral control off ART or remission, a term borrowed from cancer treatment. In this context, remission means freedom from HIV replication, as there will always be uncertainty about whether HIV levels might rebound.
The ultimate goal of a functional cure would be to enable people to stay off HIV treatment for the rest of their lives, but initially at least the aim would be to induce increasingly long periods off therapy, with periodic booster doses of the therapy that induced the remission.
Eradication of HIV from the body or long-term control of it are likely to require some combination of:
- Safe agents that can ‘wake up’ HIV in a wide range of cell types without causing dangerous inflammation (latency-reversing agents such as HDAC inhibitors).
- Therapeutic vaccines and other immune-modulating therapies that strengthen and make more specific the body’s natural immune response to HIV.
- Other drugs that can directly kill HIV-infected cells.
- Agents that can permanently disable or suppress the ability of HIV-infected cells to produce new viruses.
- Techniques that can engineer the production of cells that are resistant to infection, such as gene therapy (zinc finger nucleases or CRISPR).
Both approaches will require similar techniques, so efforts to eradicate HIV or achieve remission will support each other. Eradication might be achievable for a minority of people, but remission might be feasible for a much broader population.
Four possible paths to a cure
The rest of this page explores four broad approaches to a cure:
- Repair and replace – this includes stem cell transplants and gene editing with CRISPR-Cas9 or zinc finger nucleases.
- Kick and kill – attempts to clear the HIV reservoir, using a variety of interventions including latency reversing agents, HDAC inhibitors, PD-1 antagonists, PD-1 inhibitors, therapeutic vaccines and immune-stimulating drugs.
- Block and lock – approaches which try to trap the virus in reservoir cells so it cannot escape and replicate.
- Immunotherapies – strengthening the immune response to HIV, often using broadly neutralising antibodies.
Repair and replace
The first strategy, which we will call 'repair and replace', is the only one that has actually resulted in a cure for five people after medical intervention: the wholesale replacement of the body’s HIV-infected reservoir cells with new ones that are immune to HIV. This uses what’s still usually called a ‘bone marrow transplant’, though as the cells that essentially replace the immune system can derive from other sources, they are more usually called ‘stem cell transplants’. For reasons both of risk and expense, this strategy is never likely to be feasible for all but a handful of cancer survivors.
But there are potential ways of performing the same feat that could be less toxic and expensive. In largely preclinical and animal experiments, gene therapy has been used to snip HIV DNA out of reservoir cells; stem cells have been genetically modified to be resistant to HIV or secrete their own fusion-inhibitor-like molecules; and a therapeutic vaccine has been used to induce cells to perform the same feat.
Promising results involving snipping the DNA out of infected cells were announced as early as 2011. Modifying stem cells to produce their own fusion-inhibitor-like molecules led to decreased viral loads in monkeys in 2013.
In 2016, scientists announced the first use of a technique widely used in other areas of genetic engineering called CRISPR-Cas9 to find and remove HIV genes within human T-cells, replacing the proviral DNA with a shorter length of inactive DNA. They managed to produce T-cells resistant to HIV infection in the lab dish.
This technology however received a setback when it because clear that the proviral DNA was able to rapidly mutate to become invisible to the CRISPR guide RNA that finds the HIV DNA, and may also introduce mutations that actually facilitate this resistance. Another problem, and one common to all HIV cure approaches, is that, as in a cancer cure, the gene-editing probe would have to find and edit the proviral DNA in the vast majority of infected cells.
One way to improve the proportion of cells edited would be to create a vector. A vector is usually the shell of a virus unrelated to HIV which is good at infecting cells, but which does not replicate. Instead it acts as a mode of transport, ferrying the active drug (in this case the gene-editing CRISPR-Cas9 molecule) into the cells. Vectors are routinely used in vaccines, for instance in the Oxford/AstraZeneca COVID vaccine.
Using vectored CRISPR-Cas9 in monkeys in a study reported in 2019 appeared to clear out the proviral DNA in their cells completely. However, this was only in three monkeys and a similar experiment in China on one human subject did not edit the genes out of enough cells to result in a reduced viral load.
A combination of genetic probes may be needed to overcome resistance just as much as drug combinations are needed in ART. A study published in 2021 used CRISPR-Cas9 probes that targeted several different sequences in several HIV genes; they were introduced into cells using fat droplets containing messenger RNA, which ‘instructs’ the cells to make their own CRISPR sequences. This completely blocked HIV production in cells in the lab dish.
CRISPR-Cas9 can be used to induce cellular immunity to HIV too. A study first published in 2019 replaced the CCR5 co-receptor in a proportion of the T-cells of subjects with the mutated ‘delta 32’ version of CCR5 that confers immunity to HIV – essentially using the same technology that is central to most cases of cure with stem cell transplants, but with a much less drastic approach than a transplant. The challenge with this ‘gentler’ technology is to replace the CCR5 molecule in enough cells to confer long-lasting viral control off ART.
We are some way however from yet being able to turn gene-editing technology into an RNA vaccine (the technology used in the Pfizer and Moderna COVID vaccines) – a ‘gene shot’ that could be given to people. CRISPR-Cas9 also has potential serious side effects. Although ‘off target’ effects, which happen when the CRISPR-Cas9 probe accidentally edits human DNA rather than proviral DNA was not seen, in another Italian study it was found that some of the excised viral DNA was capable of re-assembling itself into potentially viable viral DNA, at least for a while till it is degraded by cellular enzymes.
In the meantime, results were announced in 2019 and again in 2023 from studies stretching back a decade that used an older technology – the genetic alteration of stem cells (which give rise to T-cells) outside the body using an alternative gene-editing tool called zinc finger nucleases (ZFNs) to delete their CCR5 co-receptor gene. These were then re-infused into the body. In the 2019 study the time to the reappearance of HIV in the blood was prolonged, from an average of a month to an average of three months. In the two studies announced in 2023, one produced a shrinkage of the HIV reservoir and increases in CD4 counts in nine people with persistently low counts, and in the other study, five of nine people were able to remain off ART for over a year without significant viral rebound.
Gene therapy often carries with it a note of controversy, which in the case of HIV was not helped by the notorious case of Hé Jiankui, a Chinese scientist who in 2018 claimed he had genetically altered two human embryos to make the children who were born immune to HIV. (In fact he did not produce the specific genetic alteration he wanted – the deletion of the CCR5 co-receptor for HIV.) Hé was jailed for three years for his unethical research.
On the other hand, techniques similar to the gene editing in this section have already been used successfully to treat several kinds of cancer and hereditary conditions ranging from haemophilia to spinal muscular atrophy. They are however very expensive and quite beyond the ‘target product profile’ – the combination of efficacy, cost, safety, ease of manufacture and scalability needed – for a disease affecting millions of people in the low-income world like HIV.
Kick and kill
The second strategy, often termed 'kick and kill' (or 'shock and kill'), requires less drastic immune modification than bone marrow transplants. It uses drugs to ‘wake up’ (or 'kick') the HIV reservoir cells so they become visible to the immune system (with drugs such as HDAC inhibitors), or to induce them not to go into the latent state in the first place (with drugs such as PD-1 antagonists). It was the strategy pursued most often in the early days of cure therapy, with trials of drugs aiming to flush HIV from reservoir cells starting as early as 2005.
The process whereby a proportion of cells of the immune system become quiescent and withdraw from being actively involved in immune defence is a normal part of the immune response. It enables the system to ‘remember’ infections, a feature exploited by vaccines. HIV appears to be able to induce this state in cells that would normally remain active. This phenomenon is called latency and a general name for the ‘kick’ drugs is latency reversing agents.
As first it was hoped that the body’s own immune response to these cells would slowly eliminate them, or that their differentiation back into an activated state would shorten their lives. However, these early experiments showed that only relatively small or temporary decreases in the size of the HIV reservoir resulted. So now the idea of reversing or preventing latency is now coupled with 'kill' drugs. These include therapeutic vaccines that are intended to induce a stronger and broader ‘polyfunctional’ CD8 response to the HIV-infected cells that are woken up, artificially engineered T-cells (CAR T-cells) that are tuned specifically to recognise and kill them, and investigational drugs that turn HIV’s own viral proteins against itself.
The invisibility of reservoir cells, and therefore the difficulty of developing drugs that target those cells alone, has been a problem too, as it is in the similar world of cancer therapy. Recently, gene assays that can recognise the previously invisible reservoir cells have been developed and will be an important part of the strategy.
HIV can be seen as a disease caused by a few cells that harbour disordered DNA. In this way it is similar to cancer, though in HIV the DNA is foreign – a set of instructions for making new viruses, whereas in cancer the DNA is mutated host DNA and causes cells to divide relentlessly (even if some cancer DNA mutations are caused by viruses). One of the most celebrated breakthroughs in cancer occurred in 2012 when Emily Whitehead, a girl then aged seven, was cured of leukaemia using CAR T-cells. Now CAR T-cell therapy is a recognised, if still advanced, therapy for some cancers. So, such strategies have pedigree.
Back in 2012 it was recognised that the ongoing division of reservoir cells replenished their number and that this was why HIV infection was lifelong. Some of these cells rarely if ever express HIV, but some do turn out viral particles at low levels, so it was hoped increasing the number and activation of such cells would alert the immune system to their presence. The first attempts to do this used HDAC inhibitors, already investigated as anti-cancer drugs, which cause DNA to ‘uncoil’ inside cells and thus be available for transcription (i.e. the expression of genes).
‘Kill’ in this context can mean several things. It can mean that the immune system kills off the HIV-infected cells, either directly by means of CD8 T-cells and natural killer cells, which can punch holes in infected cells, or indirectly by antibodies, which attach to the cell and alert the cell-killing machinery. But it can also involve taking the brake off processes that would otherwise lead naturally to the cell’s self-destruction by the process called apoptosis, which would normally happen in immune cells exhausted by fighting viral attack.
It was recognised early on that just relying on the immune system to control HIV reservoir cells would not be enough to eliminate them, even in cases where initiating ART very soon after infection limited the size of the reservoir. This is because releasing HIV at a rate slow enough not to unleash a new wave of HIV infection does not actually alert the immune system enough. So a large number of different therapies – combinations of broadly neutralising antibodies, therapeutic vaccines designed to stimulate antibodies or CD8 cell production, drugs such as PD-1 inhibitors that stop cells becoming part of the invisible reservoir in the first place, CAR T-cells – have all been investigated as possible ‘kill’ therapies.
But even using ‘kick’ drugs in combination with a previously administered therapeutic vaccine designed to prime an immune response to newly awoken cells did not reduce the size of the HIV reservoir in the first randomised controlled trial of a kick and kill strategy. It was an important negative result when the RIVER trial produced no reduction in the size of the HIV reservoir, even though it both increased gene expression and activation in the reservoir cells, and increased the number of CD4 and CD8 cells with HIV-specific immune activity. These two phenomena didn’t seem to link up and reinforce each other as was hoped.
Since then, there have been a number of promising studies, both animal and human, that try to eliminate the HIV reservoir or at least produce long remissions from active virus production in subjects taken off ART.
In 2022 a combination of a broadly neutralising antibody and an immune-stimulating drug, vesatolimod, enabled a group of study subjects to stay off ART for at least three months before resuming therapy, while producing one isolated case who maintained viral control off ART for four years. Around the same time, a study published in Nature found that treating 12 people with a combination of two broadly neutralising antibodies, seven on them on ART and 5 ART-naïve, resulted in five of the seven originally on ART and two of the five never on ART maintaining largely undetectable viral loads off ART for an average of 40 weeks, over four times longer than people on placebo.
Vaccines have also been designed to stimulate a more effective anti-HIV response than that seen in nature in CD4 and CD8 cells, which form the other, cellular branch of the immune system. In 2017 a therapeutic vaccine induced prolonged periods with low viral loads in five out of 13 people given it, and in 2021 the same team reported that a combination of two therapeutic vaccines produced considerably lower viral loads than viral loads prior to ART initiation in 41 subjects taken off ART for a specific period, with five maintaining viral loads below 2000.
The story of the attempt to eliminate HIV-infected reservoir cells has had as many reversals as the larger endeavour to eliminate cancers. Recent discoveries that show how challenging this aim is include finding that there may be a second reservoir of HIV-containing cells in the central nervous system; and that reservoir cells may actively keep themselves invisible by having more than the usual number of receptors on their cell surfaces for the protein PD-1, which maintains latency.
In turn, researchers have countered HIV’s cunning with MRI scans and genetic probes designed to detect the invisible reservoir cells. But it is estimated that to induce a functional cure, there may need to be a million-fold reduction in the number of cells harbouring HIV proviral DNA in the body, so our therapies to eliminate them will need to be both precise and potent.
The latest news includes the discovery that some drugs chemically related to the non-nucleoside reverse transcriptase inhibitors efavirenz and doravirine may, in addition to acting as typical ART drugs, also be able to selectively kill off HIV-infected cells. They induce the premature formation of HIV protease, which in turn helps to release cell-killing host proteins, in a process called ‘targeted activation of cell kill’ (TACK). This is a potentially exciting new ‘kill’ therapy as it does not rely on activating the immune response to HIV, but so far it has only been tested in the lab dish and reservoir cells may still need to be activated for it to work.
Meanwhile, it has been found that another class of drugs already used in cancer called BH3 mimetics or BCL-2 inhibitors may be able to specifically kill cells containing intact HIV DNA, rather than ones containing defective DNA, by ‘switching off’ a protein that confers long life to both cancer and HIV reservoir cells.
Block and lock
What if we did not need to replace or eliminate reservoir cells? What if there was a therapy that would ensure the HIV genes within them never activated, so that we could live with them and never develop a viral load?
As the kick and kill strategy is loosely based on what some elite controllers seem to do, the third strategy, 'block and lock', is based loosely on events that seem to happen in post-treatment controllers.
There are two types of post-treatment controllers. Very early antiretroviral treatment – within the first week or two after infection – sometimes allows the immune system to ‘get ahead’ of HIV’s ability to evade the body’s natural response it, producing broadly neutralising antibodies and other immune responses that stop more HIV being produced. This results in a much smaller than usual reservoir of cells containing intact proviral DNA. This strategy usually only works if people are treated very early, and it only produces long-term viral control in a fraction, such as a South African child and number of patients in France (the so-called VISCONTI cohort, the largest group of post-treatment controllers to be studied), the US and Germany.
Post-treatment control has, however, also been observed in people who start ART later, in chronic infection, in both the US and France. What may be happening here is that their proviral DNA has become inactivated due to a process of so-called ‘epigenetic silencing’. This means that the DNA in their cells has naturally adopted a form that stops the proviral HIV DNA from ever become activated: it is ‘locked’.
A similar but less conclusive process happens in a high proportion of chronically infected people. Over time, HIV reservoir cells tend to become less productive and more latent. This is because in the first few years of HIV infection, some reservoir cells are still contributing to ongoing viral replication – typically producing single-figure viral loads despite ART. In these cells, the HIV proviral DNA is often integrated into the human DNA near, or even within, the sites of frequently expressed genes.
Over time many of these cells with productive HIV gene sites will tend to undergo cell death or get picked off by the immune system. This leaves cells that only have non-viable sections of viral DNA in them, or where the genetic material is in a ‘locked’ form or located in areas that do not contain genes. The HIV DNA in these cells is maintained, or can even increase, by being duplicated, in the process of cell division, along with the human DNA – but this DNA may stay forever inactivated. This isn’t far-fetched: our genome contains genes that are there to do a specific developmental job in the womb or during childhood but are shut down forever after.
So where, in kick and kill, drugs are used to uncoil the DNA and make it active again, in theory other drugs could be used to make it never transcribable. A way would have to be found for them only to operate in cells containing HIV provirus, as drugs that unselectively block gene activity would probably be toxic. Also, for them to be cures rather than treatments, their effects on HIV DNA would have to persist for a long time, or permanently, after people stopped taking them.
A candidate drug with these properties is didehydro-cortistatin A (dCA), whose properties were originally investigated in 2012 by cure researcher Dr Nicolas Chomont. dCA inhibits HIV’s tat protein. Tat is a unique HIV product, has no human counterpart and tremendously amplifies what would otherwise be very sluggish replication. Its inhibition has been a target of a number of studies.
On ART alone, reservoir cells still produce residual amounts of HIV viral proteins. But in lab-dish experiments conducted by the Scripps institute and published in 2016, in HIV-infected cells dosed with ART and dCA and then dCA alone, the amount of residual HIV proteins they produced off ART declined to virtually zero, even when dosed with other cell-stimulating drugs. Their quiescent state persisted for months even after ART was withdrawn.
What seems to be happening is that the repeated inhibition of tat, over a period of months, leads to an accumulation of structural changes in the proviral DNA that eventually leads to a long-lasting block of its ability to produce new virus. Experiments have now been conducted in ‘humanised’ mice given ART and dCA in which viral rebound was delayed when ART was withdrawn, and the viral load after ART withdrawal was drastically reduced. Although there is a lot we do not know about exactly how dCA works, a paper published in 2021 urged human trials of dCA and also research to find drugs that may work at different stages of HIV genetic transcription to produce similar blocks.
dCA is not the only candidate for a block and lock drug. One study presented at the International AIDS Conference back in 2014 involved infecting cells in the lab dish with a messenger RNA molecule that inhibited an HIV-specific version of a protein called NF-kappa-B, which is a kinase, one of a ubiquitous family of promoters of genetic activity. Reservoir cells infected with the mRNA were resistant to activation when treated with the kind of gene-activating drugs used in kick and kill experiments.
In 2019 researchers from the University of Pittsburgh announced they were investigating 418 other diverse kinase inhibitors to find out if they too could force cells to maintain latency even in the presence of reactivating drugs. Another possibility is PD-1 agonists – drugs that boost the activity of the PD-1 molecule, which promotes latency, though they would need to be long-acting.
In 2021 a couple of reports generated more interest in post-treatment suppression of HIV. The case of the ‘Buenos Aires patient’, a woman with initially severe HIV-related illness, who was subsequently able to stop ART for, so far, 14 years without restarting was published. Another study found that only 30% of the members of the VISCONTI cohort of post-treatment controllers had had to re-start ART, and many were able to stop it again.
The new interest in block and lock as a path to an HIV cure resulted in 2022 in the launch of a global research consortium called HOPE which stands for HIV Obstruction by Programmed Epigenetics.
The lack of HIV activity in post-treatment controllers might be due to two processes reinforcing each other. The long-term development of deeper latency and genetically blocked HIV DNA could reduce inflammatory responses and in turn, the activation of even fewer cells.
This implies we might be able to get to a ‘benign circle’ situation where, whether the death of actively HIV-infected cells or epigenetic silencing happens first, we could live healthily off ART with a residual amount of HIV. The two strategies might be used sequentially – 'kick and kill’ to eliminate all but the last few HIV-infected cells, and ‘block and lock’ to prevent any activation of the tiny number still left. In the long term, both strategies could lead to a functional cure.
Immunotherapies
The 'kick and kill' and 'block and lock' strategies aim in the first place to shrink the reservoir of cells actively infected with intact proviral DNA, but it is implied that some kind of immune response to HIV will be needed to cull or inactivate the small number of infectious cells that may remain.
In addition, a more ambitious goal of cure research is to find an immune response to HIV that it sensitive, selective and potent enough to do the whole job of targeting and killing the reservoir cells, and only them.
The problem for both cure and vaccination strategies for HIV is the body cannot – except in very rare cases of elite control – mount an immune response capable of clearing HIV infection naturally. This is unlike the majority of infections, where the immune system can do this: if it did not, COVID-19 would be as lethal as AIDS.
In order to cure HIV, therefore, immunotherapies have to do ‘better than nature’, and also have to get around the problem that the inflammatory response to HIV – the immune defence against it – only increases the number of T-lymphocytes and other immune cells for HIV to infect.
We do have an example of immune responses to HIV that could be harnessed as part of a cure in the shape of broadly neutralising antibodies (bnAbs).
Antibodies are small Y-shaped protein molecules that are produced in profusion by B-lymphocytes in response to many varieties of foreign invader – viruses, bacteria, drugs, pollutant particles, and in the case of auto-immunity, even the body’s own tissues. They directly neutralise invaders by physically blocking them from doing damage, but also act as ‘distress flags’ signalling to other parts of the immune system.
Antibodies are produced in response to HIV infection and are what are detected in standard tests for HIV diagnosis. They are even capable of neutralising certain, less virulent strains of HIV – as was shown by the weak immunity seen in the RV144 vaccine trial.
This antibody response is insufficient to contain HIV, however. This is partly because HIV conceals its most ‘conserved’ (i.e. essential) structures beneath a wrapping of sugar molecules and by having envelope proteins that do not unfold into the correct shape until seconds before infection. And it is also because HIV is such an immensely variable virus that it can easily outpace the immune system’s ability to generate antibodies against each new strain.
However, in about 15% of people with chronic HIV infection, bizarre, hypermutated antibodies eventually develop with ‘prongs’ that can get behind HIV’s defences and neutralise large numbers of different strains (hence ‘broadly neutralising’). In chronic infection these develop far too late to make a difference – HIV has already evolved beyond them.
However, bnAbs can be used as sophisticated biologic drugs when harvested from the blood of those who have them and then cloned in bulk. (The cloning process explains why they are sometimes described as monoclonal antibodies.) They already have a firm place in modern cancer therapy. One of their advantages is that a single infusion of long-acting versions of them can last several months.
BnAbs do not only act passively as HIV entry blockers. Because they also recruit other parts of the immune response, such as the CD4 and CD8 responses that can kill off infected cells, they can produce longer-term viral suppression off ART.
We only have room here to present a couple of the more promising strands of research into HIV immunotherapies – many more are being investigated.
In 2018, a study using two bnAbs called 3BNC117 and 10-1074 enabled two out of 11 trial subjects to stay off ART with undetectable viral loads for at least seven months (the length of the study).
In 2020, another study using just one of the same antibodies (3BNC117, plus or minus an immune-stimulant drug, romidepsin) resulted in seven out of 59 participants being able to stay undetectable off ART for at least three months. One participant appeared to be in long-term remission, with viral suppression off ART that had lasted, at the time of the report, for 3.7 years, and with HIV RNA and DNA levels that continued to decline. An ongoing problem for bnAbs as a cure or maintenance therapy is that many strains of HIV have pre-existing resistance to specific bnAbs and in this trial, 12 out of 31 participants given the two antibodies had resistance to one or both of them and derived little benefit. It is an ongoing debate as to whether trial candidates should be screened for antibody resistance before enrolment.
A sign that this strategy was being taken seriously by ‘big pharma’ was when Gilead Sciences started a study called TITAN of the 3BNC117 and 10-1074 antibodies, which have now received generic names: teropavimab and zinlirvimab. These were given with or without Gilead’s immune boosting drug lefitolimod to 24 people. Two of them had maintained viral suppression off ART for 18 months at the time of the study’s publication in September 2023.
For longer-term dosing, antibodies can also be introduced with viral vectors, as a form of vaccine that induces the immune system of the recipient to make its own antibodies. These have been used in animal studies to cure several monkeys. Genes selected from the B-lymphocyte cells that make specific antibodies were packaged inside a vector (a shell of a non-pathogenic virus that could infect other cells but not replicate further). These induced the monkeys to make more antibodies, producing long periods of viral suppression off ART, in one monkey out of four in a first study, and in three out of eight in a second.
Even if viral resistance to antibodies is circumvented, the immune system of the recipient can develop its own antibodies against the ‘foreign’ antibodies, even if introduced with a vaccine. This is why eight out of 12 monkeys in the two studies above did not respond.
‘Anti-antibodies’ and resistance are both less likely to happen if a vaccine induces the production of high levels of a number of variants in a process that more closely mimics the naturally diverse reaction to an infection. To overcome this, it is becoming possible to make vaccines that will induce the body to make its own bnAbs in advance of infection. While these will function primarily as prevention medications, an immune response of sufficient diversity and potency could also act as part of a cure.
The most advanced vaccines currently being tested aim to induce the stem cells in recipients’ bone marrow – which are capable of turning into any kind of cell – to develop into B-lymphocytes capable of generating bnAbs. Two studies in 2022 and in 2023 have now demonstrated that this approach is possible, though we are at an early stage in this strategy.
The antibody response is not the only immune system response that might contribute to a cure. In a study in 2012, 50% of a group of 96 monkeys were cured of HIV infection with a revolutionary ‘replicating vector’ design that stimulated a broad and varied response by the other part of the adaptive immune system, the cellular part, which largely consists of CD4 and CD8 cells.
This vaccine had been intended to prevent HIV infection but instead, and unprecedentedly, it acted after infection, producing progressively lower viral loads and other signs of viral activation in the monkeys until they appeared to be functionally cured. The prior vaccination generated a strong and uniquely diverse CD8 response to HIV, which did not shrug off subsequent infection, but instead slowly eliminated it after infection had initially happened. Hence its interest for cure research: the strategy is being pursued in human studies, although so far with limited success.
This is not the only or even the most advanced study of attempts to induce a cure using the CD4/CD8 response. A Spanish study published in 2021 using varieties of HIVACAT, a length of HIV protein designed to induce a cellular immune response, gave two different varieties of it as a therapeutic vaccine sequentially to 30 volunteers. Eight recipients maintained low viral loads (defined as lower than 10,000) for 24 weeks after coming off ART.
Gilead Sciences also picked up on this research and supported the next trial, whose interim results were reported in 2023. Thirty-three people were given the HIVACAT-based vaccines, plus or minus vesatolimod. Thirty agreed to stop their ART and of those 10 (33%) were able to stay off ART for the three months maximum in the study protocol. (It’s notable, though, that four (24%) of the 17 placebo recipients in the study also managed to do this.)